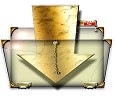
Spheroids were then incubated at 37 ☌ with 5% CO 2 for further analysis. Thermal increase was monitored over time by focusing a thermal camera (Optris) on the irradiated spheroids. The irradiation of cells was performed for 5 min at a power density of 6 W/cm 2. The spot of the laser had a diameter of 0.8 cm. To perform photothermal therapy, spheroids were transferred at every timepoint under an 808 nm laser (Laser Ever). The nanomaterial was directly drop casted upon the ATR crystal and the spectra were recorded in the wavenumber range of 4000–550 cm −1. Chemical analysis of the nanoparticles was performed using attenuated total reflectance-Fourier transform infrared spectroscopy (ATR-FTIR) by Spectrum One spectrometer (Perkin Elmer, Waltham, MA, USA). Data analysis was performed by Malvern Zetasizer software. The hydrodynamic radius (Z-average size) was obtained by using the Stokes−Einstein equation. The measurements were performed at a fixed position (4.65 mm) with an automatic attenuator. UV-transparent cuvettes (Malvern, Herrenberg, Germany) were used for experiments with a sample volume of 500 μL and a concentration of 100 μg/mL. Dynamic light scattering was performed with Zetasizer Nano ZS (Malvern, Herrenberg, Germany), equipped with a 633-nm He−Ne laser and operating at a scattering angle of 173°. Images were acquired using silicon cantilevers with conical silicon tips (CSC36 Mikro-Masch, Tallinn, Estonia) characterized by an end radius of about 10 nm, a half conical angle of 20°, and a spring constant of 0.6 N/m. Then, 100 μL of samples with a concentration of 10 μg/mL was deposited on sterile mica slides and air-dried overnight for atomic force microscopy imaging (AFM) with a NanoWizard II (JPK Instruments AG, Berlin, Germany). Fluorescence intensity spectra were normalized to the maximum emission. Fluorescence intensity spectra were obtained by using a Cytation 3 Cell Imaging Multi-Mode Reader (Biotek, Terrebonne, QC, Canada), using excitation wavelengths from 260 to 600 nm and acquiring emission from 300 to 700 nm. Optical and spectroscopic characterizations of GQDs were carried out as described elsewhere.
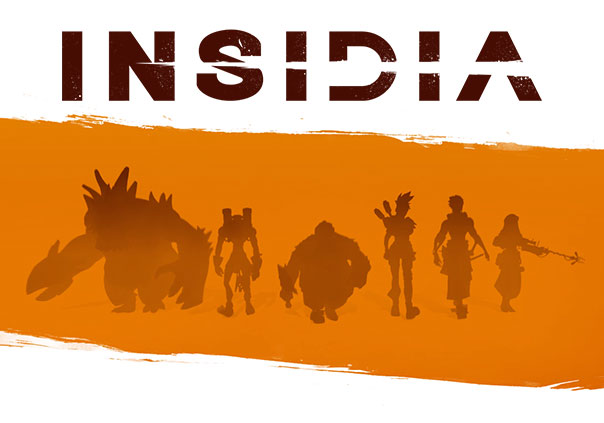
We have summarized these effects in a quantitative parameter of spheroid disruption demonstrating that INSIDIA 2.0 multiparametric analysis can be used to quantify cell death in a non-invasive, fast, and high-throughput fashion.Ĭarboxylated GQDs in double-distilled water (ddH 2O) solution with a concentration of 1 mg/mL have been purchased from ACS Material (Pasadena, CA, USA). On the other hand, PANC-1 spheroids’ death caused by nanoparticle photothermal disruption is accompanied with an overall increase in area and entropy due to the progressive loss of integrity and increase in variability of spheroid texture. Thanks to INSIDIA 2.0 analysis, two types of effects have been observed: In U87 spheroids, death is accompanied by a decrease in area of the entire spheroid, with a decrease in entropy due to the generation of a high uniform density spheroid core. INSIDIA has been implemented to provide in-depth morphologic analysis and has been used for the analysis of the effect of graphene quantum dots photothermal therapy on glioblastoma (U87) and pancreatic cancer (PANC-1) spheroids. In this work, we report the INSIDIA 2.0 macro, which offers researchers high-throughput and high content quantitative analysis of in vitro 3D cancer cell spheroids and allows advanced parametrization of the expanding and invading cancer cellular mass.
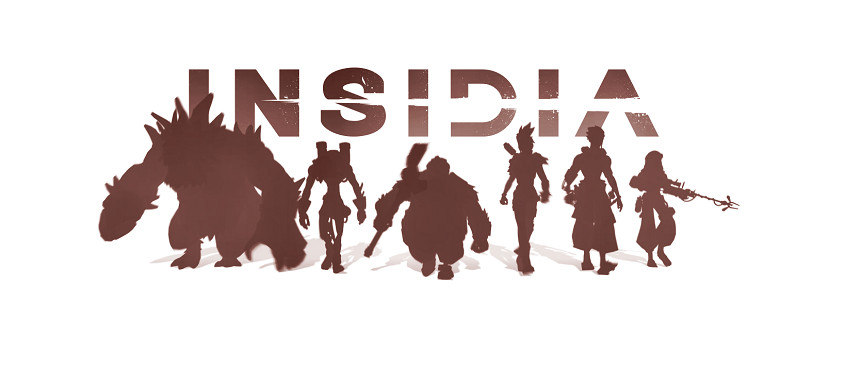
INSIDIA MAP SOFTWARE
For this reason, rapid, user-friendly, ready-to-use, high-throughput image analysis software is necessary. This is a time-consuming process and is extremely liable to the variability of individual operators.
INSIDIA MAP MANUAL
However, most of the current spheroid analysis methods involve manual steps. Cancer spheroids are in vitro 3D models that became crucial in nanomaterials science thanks to the possibility of performing high throughput screening of nanoparticles and combined nanoparticle-drug therapies on in vitro models.
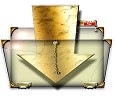